![]() |
|
||||
BiographyAng Feng was born and raised in Guizhou, China. He specialized in materials physics and chemistry and received his master’s degree from the University of Chinese Academy of Sciences. In May 2022, he earned his Ph.D. in physics from Ghent University for his thesis on charge trapping/detrapping in luminescent materials. Since January 2023 he has been with the Institute for Microelectronics as a postdoctoral researcher, where he focuses on the reliability of SiC MOSFETs. |
Leakage Current in SiC Power MOSFETs
Electric vehicles (EVs) are gaining momentum in the automotive industry due to efficient energy utilization and stringent environmental regulations. The Silicon Carbide (SiC) metal-oxide-semiconductor field-effect transistor (MOSFET) is one of the most promising semiconductor devices for power control and energy management of EVs. SiC power MOSFETs offer higher efficiency, higher power density, and smaller device sizes than their Silicon counterparts.
SiC MOSFETs often operate at high dielectric fields to further minimize the ON resistance by enhancing the channel density. Unfortunately, the carriers can tunnel into the dielectric (e.g., SiO2) via the Fowler-Nordheim (FN) mechanism. The resultant leakage current and charge capture can cause device reliability issues, e.g., bias temperature instability. At even higher fields, the tunneling carriers become so energetic (‘hot’) that they can trigger impact ionization, accelerating device degradation and breakdown. These concerns are more pronounced at lowered temperatures because of enhanced impact ionization. Hence, modeling the transport of hot carriers (leakage currents) is crucial for evaluating the reliability of SiC MOSFETs.
Traditionally, the complete solution of the 6-dimensional (6d) Boltzmann transport equation (BTE) has been employed for this purpose, albeit at a significant computational cost. In response, we propose a simplified one-dimensional (1d) BTE, which incorporates electron-phonon scattering and impact ionization into the relaxation length λ(E, T) as a parameter. It can provide a good approximation for the evolution of hot carriers’ energy distribution function (EDF), enabling facile calculation of the leakage current and impact ionization rate. The 1d BTE was implemented within the Comphy simulator that handles the electrostatics of the device (see Fig. 1).
As an example, the solution of 1d BTE was obtained for a SiC-SiO2-Si capacitor. The spatial evolution of EDFs clearly shows the formation of high energy tails [E>Eg(SiO2)] that are a precondition for impact ionization (Fig. 2 left). They also exhibit the ‘thermal tails’ due to carriers’ ballistic transport. There are also interesting features in the electron multiplication factor m(x) (Fig. 2 right) and the associated impact ionization rate coefficient nII(x) (Fig. 2 middle). The gate leakage current now includes the amplification due to impact ionization.
In summary, a model has been developed to describe impact ionization and its influence on the gate leakage current for the dielectrics of power MOSFET operating under high fields.
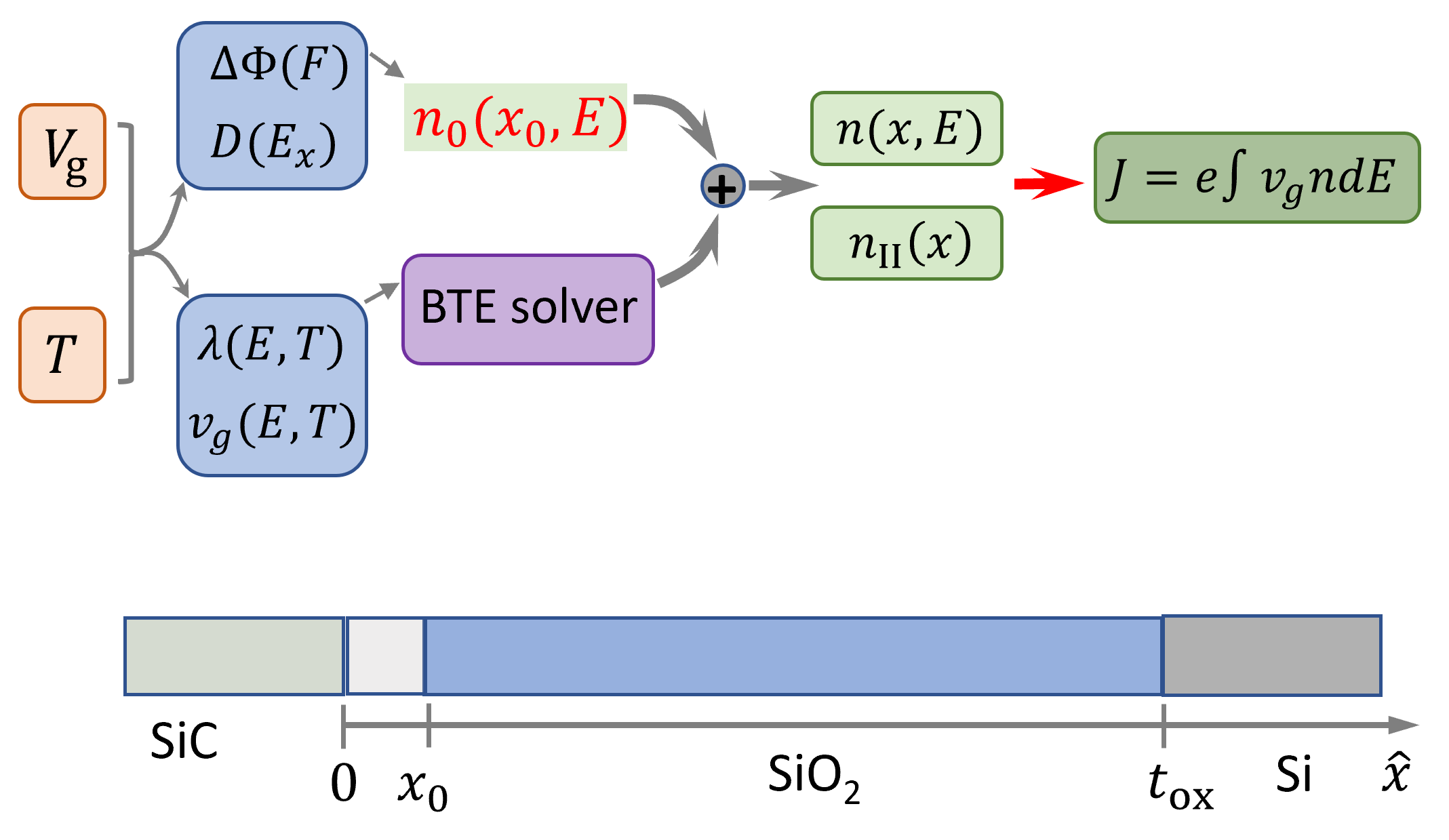
Fig. 1: The 1d BTE integrated with the Comphy simulator. The Comphy simulator handles the electrostatics (field, potential, etc.) and the FN tunneling at operation temperature T and bias Vg. The 1d BTE determines the evolution of EDFs along the dielectrics (e.g., SiO2), given the relaxation length λ(E, T). The impaction ionization rate can thus be obtained from these EDFs.
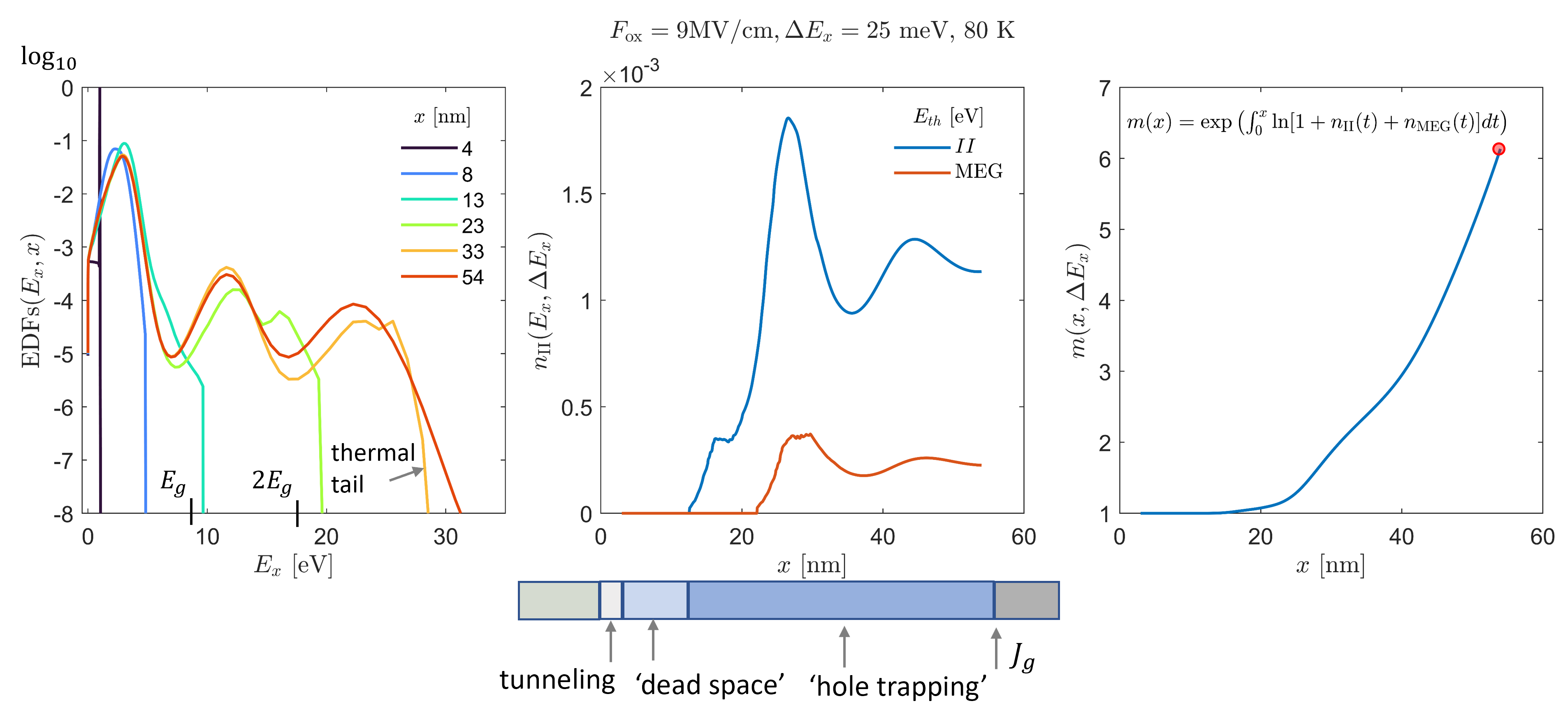
Fig. 2: The evolution of EDFs and impact ionization rate along the insulator. The 1d BTE solution was obtained for a SiC-SiO2-Si capacitor under 9 MV/cm at 80 K. The spatial evolutions of EDFs indicate high energy tails for impact ionization (left). The simulated electron multiplication factor m (right) and the impact ionization rate coefficient (middle) show interesting features.