![]() |
|
||||
BiographyBernhard Pruckner was born in 1994 in Vienna. He received his BSc degree in Technical Physics at the TU Wien in 2017. After completing his BSc degree, Bernhard was offered an exchange internship at Instituto Superior Tecnico in Lisbon where he was modeling laser-plasma interactions using a finite difference method. He continued his study at the TU Wien simultaneously working on his Master thesis at the Austrian Institute of Technology on coupled finite volume - finite element approach for simulating solidification processes in aluminum alloys. He successfully defended the thesis at the TU Wien in 2020 and received his Masters degree in Technical Physics. After working at the Division of Nuclear Medicine at AKH Wien he joined the Institute for Microeletronics in December 2022, where he is pursuing a PhD degree in micromagnetic simulations for non-volatile magnetic memory devices. |
Magnetic Spin Hall Driven Field-Free Magnetization Switching of SOT-MRAM Devices
Spin-orbit torque magnetoresistive random access memory (SOT-MRAM) has been established as a promising non-volatile memory technology, offering high switching speed, low power consumption, and long endurance due to the separation of the read and write paths. An MRAM cell core consists of a magnetic tunnel junction (MTJ) made up of two ferromagnetic (FM) layers separated by a tunnel barrier (TB), which sits on a heavy metal (HM) layer, as shown in Figure 1a. The magnetization of the FM reference layer (RL) is fixed. In contrast, the FM-free layer (FL) magnetization is variable and can be manipulated by external magnetic fields or spin currents. The MTJ can assume two different states, corresponding to the logical states 1 and 0, depending on the relative orientation of the magnetization in the FL and RL. The electrical resistance of the MTJ is significantly higher in the antiparallel state than in the parallel state due to tunneling magnetoresistance (TMR), allowing for easy reading of the two states.
To alter the state of the cell, a write current is applied in the HM layer along the x-direction. Spin currents are generated inside the HM and at the HM/FM interface through bulk and interface effects, respectively. The primary contribution to spin current generation comes from the spin Hall effect (SHE) in the bulk of the HM. Perpendicular-to-plane spin currents are generated and injected into the adjacent FL, where they are quickly absorbed (Fig. 1a). The conservation of momentum results in a torque on the magnetization that corresponds to the absorbed spin current, enabling efficient manipulation of the magnetization. The SOTs generated by the SHE act to bring the magnetization of the FL in-plane. This poses a challenge for the design of SOT-MRAM with perpendicular magnetization. For deterministic out-of-plane switching, the SHE-generated SOTs require assistance, which can be achieved by applying an external magnetic field along the direction of the charge current. However, this method restricts the scalability and complicates the circuit architecture of the device. Therefore, methods to generate out-of-plane spin-polarized spin currents in SOT-MRAM have been the subject of intensified research.
The magnetic spin Hall effect (MSHE) in antiferromagnetic (AFM) materials can overcome this limitation and achieve field-free SOT switching. This different type of SHE results in an anti-damping-like torque that acts to switch the magnetization out-of-plane (Fig. 1b). The switching of a perpendicularly magnetized FM layer adjacent to an MSHE-layer has been observed in multilayer structures with a non-collinear AFM Mn3Sn layer as a source of these unconventional spin currents. Spin currents and torques generated by the SHE and the MSHE in an HM/FM and AFM/FM bilayer, respectively, are depicted in Figure 2c and Figure 2d. We employ a fully three-dimensional finite element method (FEM) based simulation approach, which couples the charge and spin currents with the magnetization dynamics, to evaluate the physical phenomena responsible for the magnetization switching of SOT-MRAM devices. We model the magnetization dynamics with the Landau-Lifshitz-Gilbert (LLG) equation. We use a coupled spin and charge drift-diffusion (DD) formalism to describe the distribution of the spin accumulation. SOTs are included by considering the spin-charge angle (SCA) tensor reported for Mn3Sn non-collinear AFM. The SCA describes the charge-to-spin conversion and generation of additional out-of-plane spin-polarized spin currents, which lead to anti-damping-like torque in the device's FL that can push the magnetization out-of-plane without the support of an external field. A comparison of SHE-driven switching with and without an external magnetic field and MSHE-driven switching of a SOT-MRAM device with an initial out-of-plane magnetization is shown in Figure 2.
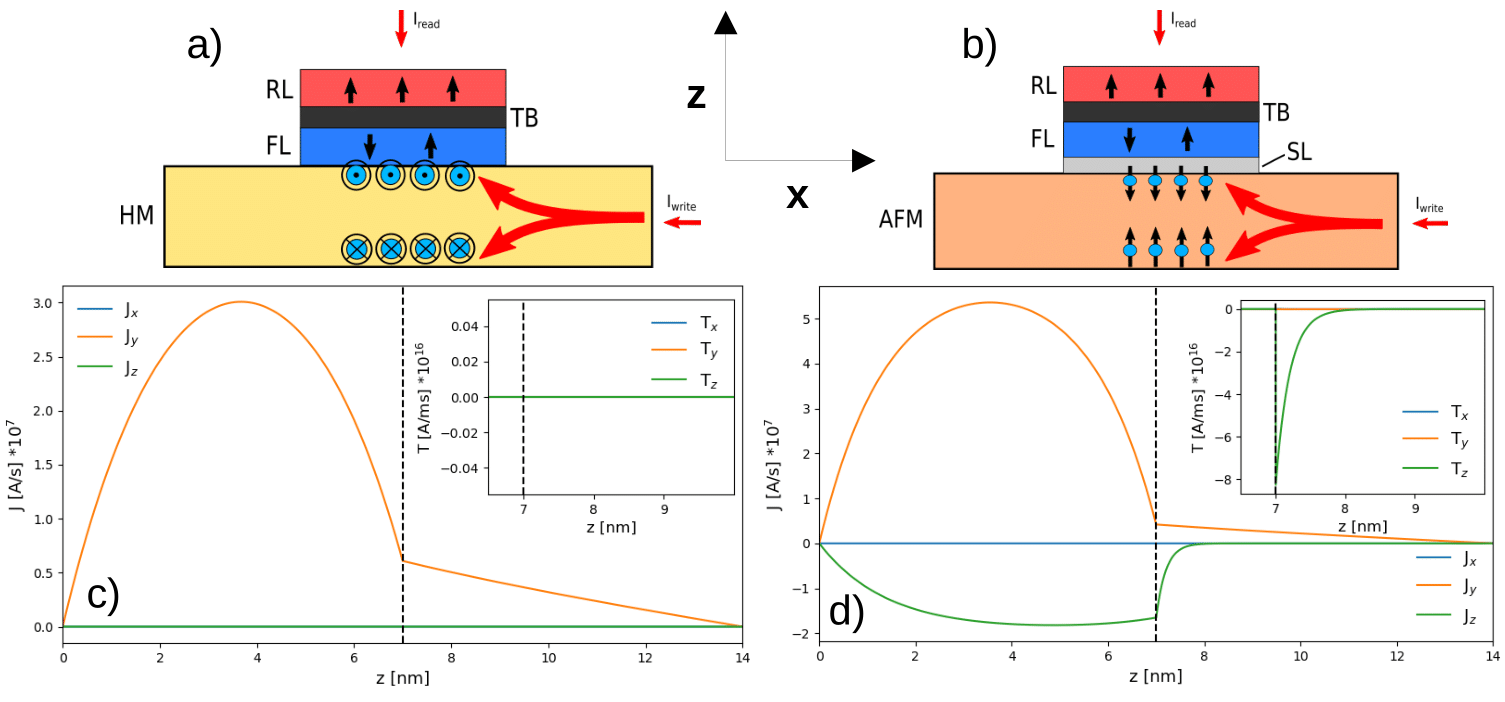
Fig. 1: Schematic view of a SOT-MRAM device utilizing the SHE (a) and the MSHE (b). The SHE produces spin currents with in-plane spin polarization, which impinges on the adjacent magnetic free layer (FL) and pushes the magnetization in-plane. The MSHE produces spin currents with out-of-plane spin polarization, which pushes the magnetization of the FL out-of-plane and enables full perpendicular magnetization switching. A non-magnetic spacer layer is inserted between the antiferromagnetic layer and the FL in (b) for magnetic decoupling of those layers and to ensure that spin currents solely drive the switching process. The spin-currents and torques in an FL with in-plane magnetization produced by SHE and MSHE are shown in (c) and (d).
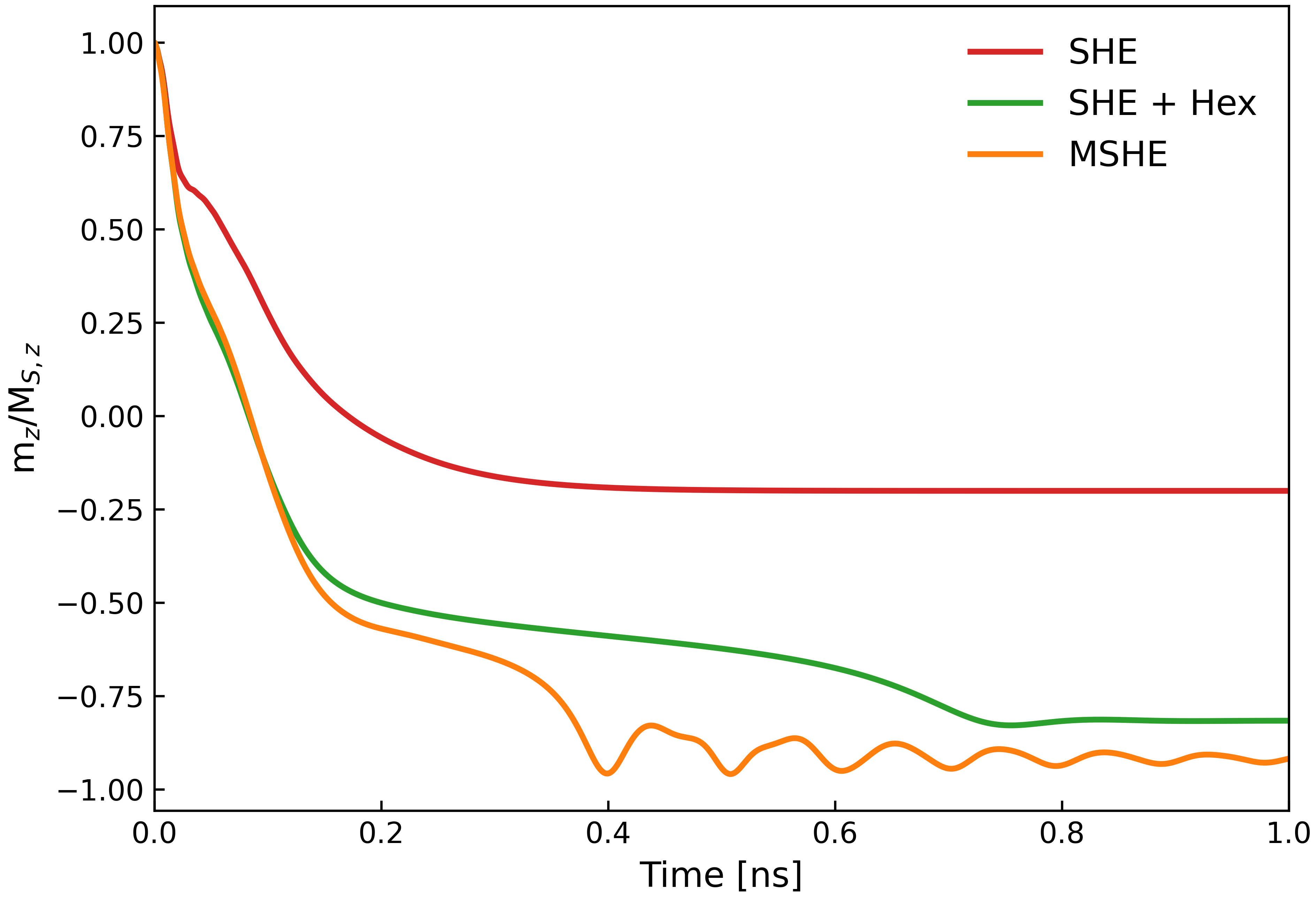
Fig. 2: The normalized z-component of the magnetization of the FL during perpendicular magnetization switching. While SHE only acts to bring the magnetization in-plane, an additional external field (Hex) acts to complete the perpendicular switching. The spin currents produced by MSHE lead to complete perpendicular magnetization switching without using an external field.