![]() |
|
Biography
Paul Ellinghaus was born in 1986 and grew up in Pretoria, South Africa, where he completed his Bachelor of Electronic Engineering, at the University of Pretoria, in 2008, whereafter he worked as a research engineer for the university spin-off company, INSiAVA, for 9 months. He completed his master's studies in mathematical engineering, within the Erasmus Mundus MathMods consortium, in L'Aquila (Italy) and Nice (France), in 2011. Thereafter, he joined the process integration group at IHP Microelectronics, in Frankfurt (Oder), Germany, as a research assistant for 15 months. Since January 2013, he is at the Institute for Microelectronics, supporting research efforts relating to the Wigner-Boltzmann equation, in pursuit of his doctoral degree.
Wigner Monte Carlo Simulation Moving Towards Applications
Significant progress has been made in further developing the signed-particle Wigner Ensemble Monte Carlo simulator, forming part of the ViennaWD suite of simulation tools during the past year. The simulator can now handle two dimensional domains, classical scattering mechanisms and is able to reliably simulate quantum evolutions over several hundred femtoseconds, which allows steady-state solutions to be found. These achievements have been made possible by, first, uncovering and treating idiosyncrasies of the particle generation and annihilation algorithms and, second, a distributed-memory (MPI) parallelisation of the code to handle the computational load.
The semi-discrete Wigner equation considers a finite range of discrete momentum values. The quantum nature of the evolution is captured by the generation of signed-particle pairs with a momentum offset. Generating these offsets in symmetric pairs has been shown to gradually bias the statistics. As a result, this issue has been solved by generating the momentum offsets independently and revising the acceptance/rejection criteria for what forms a valid particle. The regeneration of particles, after an annihilation step, was found to introduce an artificial numerical diffusion, which can be counteracted by calculating the pre-annihilation mean of the particle ensemble in each spatial cell.
The consideration of two spatial dimensions and three dimensional wave vectors dramatically increases the size of the phase space, which increases the memory requirements to represent it and also the number of particles required to resolve the Wigner function with a reasonable level of statistical confidence. A spatial-domain decomposition approach has been adopted, implemented using MPI, to allow the computational burden and memory requirements to be spread over multiple nodes in a distributed-memory, high-performance computation environment.
The activities of the past year bode well for the application of the Wigner Monte Carlo simulator to take on increasingly complex problems with practical application, e.g., Fig. 1 shows how periodically injected wave packets can be focused into a nanoscaled channel using an electrostatic lens, which increases the steady-state current through the channel.
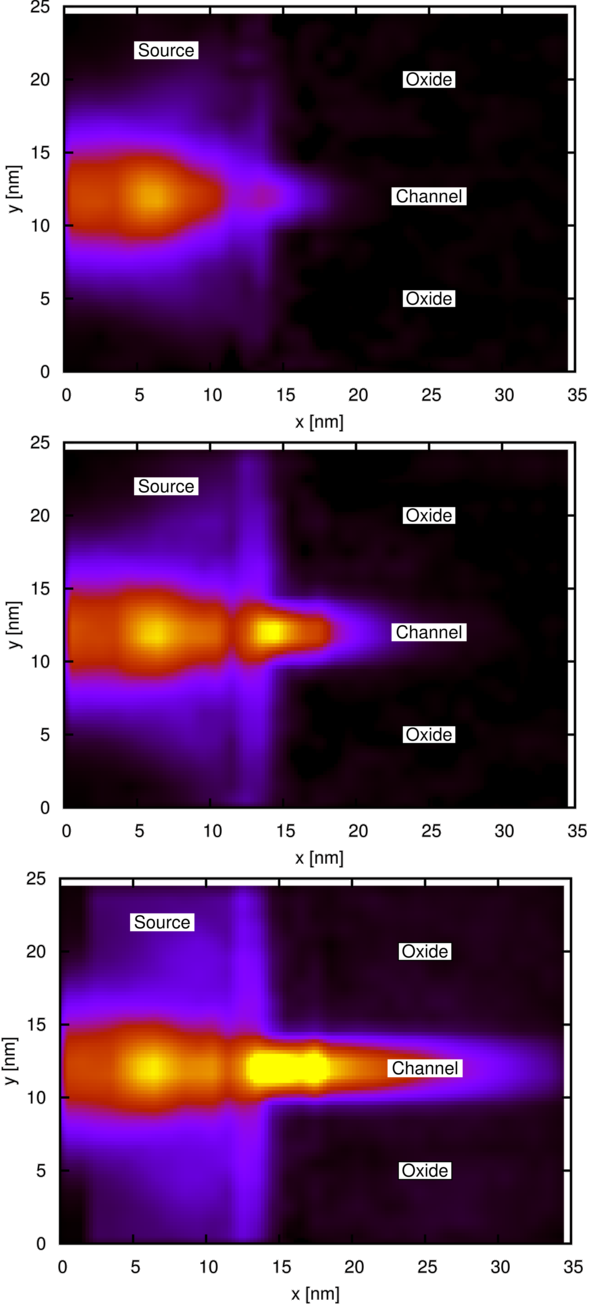
Fig. 1: Electron density at 40fs, 50fs, and 70fs. Wave packets are periodically injected from the left boundary and are focused into a 5nm wide channel by an electrostatic lens in the source region.