![]() |
|
Biography
Dr. Mischa Thesberg is a Canadian researcher within the Institute for Microelectronics. He has a BSc (2008) in computational science from the University of Waterloo and an MSc (2010) and PhD (2014) in computational condensed matter physics from McMaster University. His research focuses on quantum transport and high-performance computing methods and his most recent interests include thermoelectrics, two-dimensional materials, novel nanoelectronics devices and quantum transport formalisms such as the non-equilibrium green's function approach.
NEGF Studies for Power Factor Optimization of Energy Filtering Thermoelectrics
Waste heat is created everywhere; in manufacturing, in the engine of an automobile, in the production of power, in the operation of computer chips, and in countless other places. The potential to turn even a fraction of this loss into useable energy is tremendous. Although heat engines can have efficiencies in the range of 30%, they have clear limits to their application: They cannot be scaled down in size, they must be actively maintained, and their operation is complex with moving-parts and fluids. The result is that many forms of waste heat have traditionally been considered "unrecoverable".
A thermoelectric material is able to drive a current when an external temperature gradient is applied. Its ability to do so is a property of the material itself and therefore thermoelectric generators have no mechanical parts, require no maintenance, and can even be scaled down to recover heat from small sources, like computer chips. Thus, thermoelectrics have the potential to fill a niche in waste heat recovery and thus green technology that has not been traditionally met. However, the field of thermoelectrics lies on a sort of precipice; although many theoretical schemes for engineering efficient thermoelectrics exist, commercially available thermoelectrics are still too inefficient for most applications.
The effectiveness of a thermoelectric material's energy conversion is quantified by the figure of merit, ZT. State of the art research thermoelectrics have figures of merits of approximately ZT = 1. However, it is estimated that ZT > 3 is required in order for thermoelectric materials to be a viable energy technology beyond niche applications. Thus, the need to improve the figure of merit is paramount.
Many of the most popular approaches aimed at increasing the figure of merit revolve around finding the ideal material with maximum ZT. However, other schemes involve explicitly constructing nano-sized features in more mundane materials in order to engineer thermoelectric improvement. There exists no material that can be more accurately and cheaply nano-engineered than silicon. Silicon, however, is typically a very poor thermoelectric, with a ZT ~ 0.01, though it has been shown that nano-engineering can improve that figure by at least an order of magnitude.
A promising avenue towards improvement of silicon's thermoelectric efficiency is through the introduction of a grating of potential barriers into the system. Such barriers could be the result of nanogranularity, with the grain boundaries acting as barriers, or through the creation of a superlattice. It has been suggested that such a set-up could vastly improve silicon's ZT. However, the effectiveness of such an approach is likely dependent on a level of precision in the shape and size of these barriers.
Using the Non-Equilibrium Green's Function (NEGF) approach (sample data can be found in Fig. 1) we have been studying both the effects that the shape and size of these barriers have on ZT, and the amount that the improvement is robust against random variation and non-idealities in the barrier grating. It is important both to understand how effective these barriers can be and how feasible their use is within the context of a real system. The NEGF work includes scattering from both acoustic and optical phonons, and the energy exchange brought by such phonons plays a crucial role in the physics.
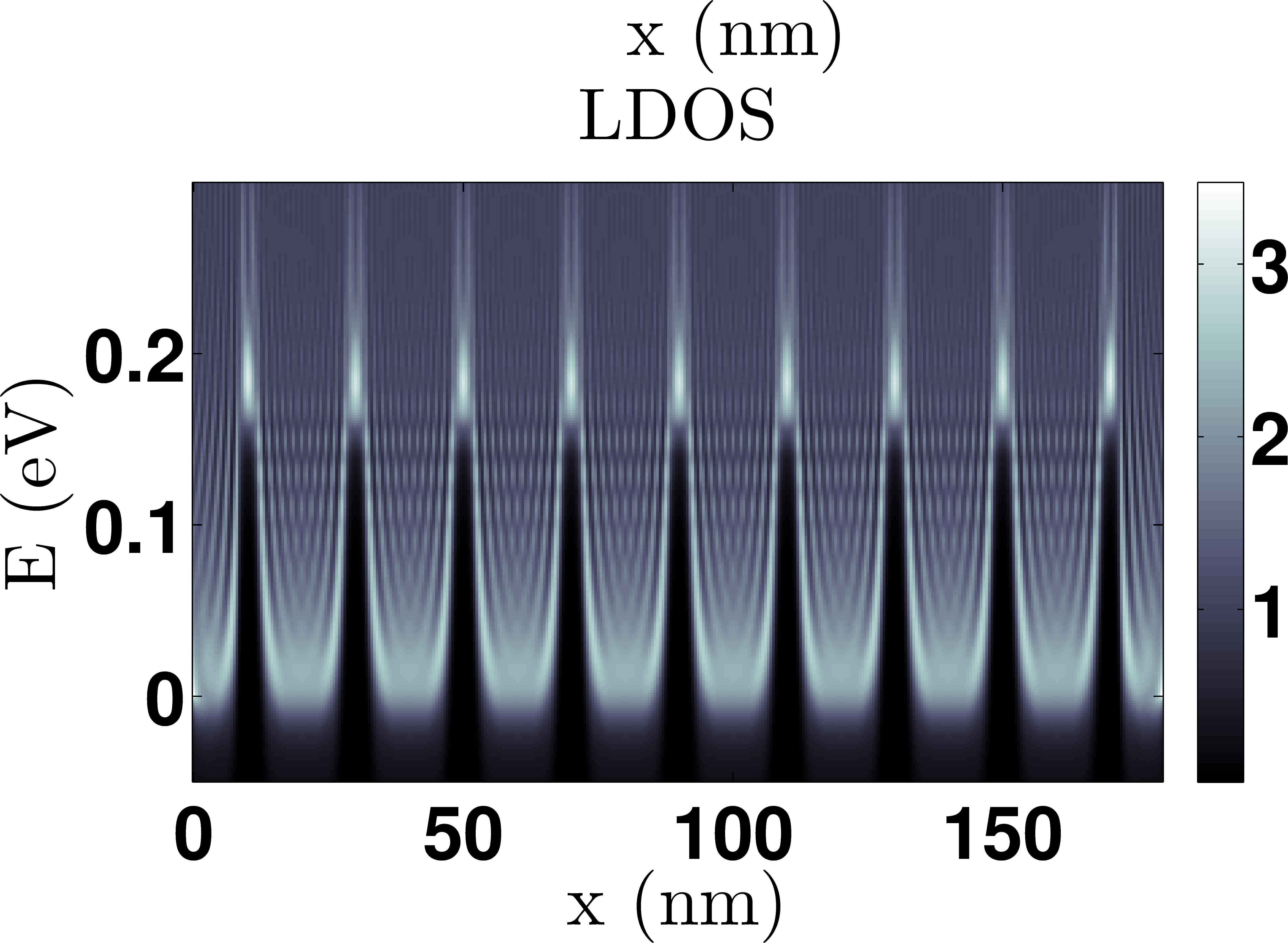
Fig. 1: A sample Local Density of States (LDOS) of a one-dimensional superlattice of energy filtering potential barriers.