![]() |
|
Biography
Wolfgang Gös was born in Vienna, Austria, in 1979. He studied technical physics at the Technische Universität Wien, where he received the degree of Diplomingenieur in 2005. In January 2006, he joined the Institute for Microelectronics and focussed on modeling of the bias temperature instability. In 2007, he was a visitor at the Vanderbilt University in Nashville, TN. In 2011, he received his doctoral degree and currently holds a post-doc position at the Institute for Microelectronics, where he continues his research activities in reliability issues of semiconductor devices. His current scientific interests include atomistic simulations, the chemical and physical processes involved in NBTI and HCI, and reliability issues in general.
Statistical Analysis of Defect Parameters
During the last years it has been revealed that Bias Temperature Instability (BTI) degradation is dominated by the capture and emission of charge carriers from the substrate. Therefore, these two processes have been intensively investigated within the framework of the multi-state Nonradiative Multi-Phonon (NMP) model. In this model, capture and emission times are linked to defect parameters, such as microscopic energy barriers and trap levels, which can be deduced from their Potential Energy Surface (PES). Since the defects are in an amorphous host material, their PESs and in consequence also their capture and emission times vary dramatically from defect to defect. As such, it is of high importance to determine the statistical distributions of the PES variations. This goal is pursued using three different methods:
First, the PESs of defects are computed at special points, such as equilibrium configurations and the maximum of barriers, using first-principles calculations. These simulations are performed at numerous different sites within an aSiO2 supercell for a handful of defect candidates which are suspected to be responsible for BTI (the hydrogen bridge, the hydroxyl E' center and the oxygen vacancy). Interestingly, our Density Function Theory (DFT) calculations predict that a considerable number of these defect structures are stable in two configurations as required by the mutli-state NMP model.
Second, the degradation curves of a large-area transistor are recorded for different combinations of stress voltages and device temperatures. Then the multi-state NMP model, which has been complemented by a double-well model to capture the permanent component of BTI, is calibrated against the recorded degradation using 3,600 representative traps. As this device contains a large number of defects, this method is well suited for the extraction of distributions of trap parameters.
Third, the defect parameters are obtained by fitting the multi-state NMP model against the capture and emission times of single defects obtained by Time-Dependent Defect Spectroscopy (TDDS). Even though TDDS allows for the investigation of single defects in unprecedented detail regarding their gate bias and temperature behavior, this approach only provides the parameters for a handful of defects.
The result of the three methods were compared in histograms and correlation plots to investigate the distribution of the defect parameters. The most decisive parameters are the thermodynamic trap level and the energy barrier between the two stable configurations in the multi-state NMP model, both shown in Fig. 1 and Fig. 2, respectively. The defect parameters extracted from TDDS and the measured degradation curves yield a trap level slightly below the valence bandedge while the hydrogen bridge and the hydroxyl E' center tend to have lower values in aSiO2. Even though the mean values of the trap levels slightly deviate, the obtained distributions are still in a good agreement due to their large overlap. The barrier heights calculated from experiments were found to be centered around 1eV. However, the DFT calculations of the two defect candidates predict a range of energy barriers up to 1.25eV. Still, the obtained distributions allow for a sufficient large number of defects with the sought defect property. From this, it can be concluded that both defect types are promising candidates, with the hydroxyl E' center being slightly favored.
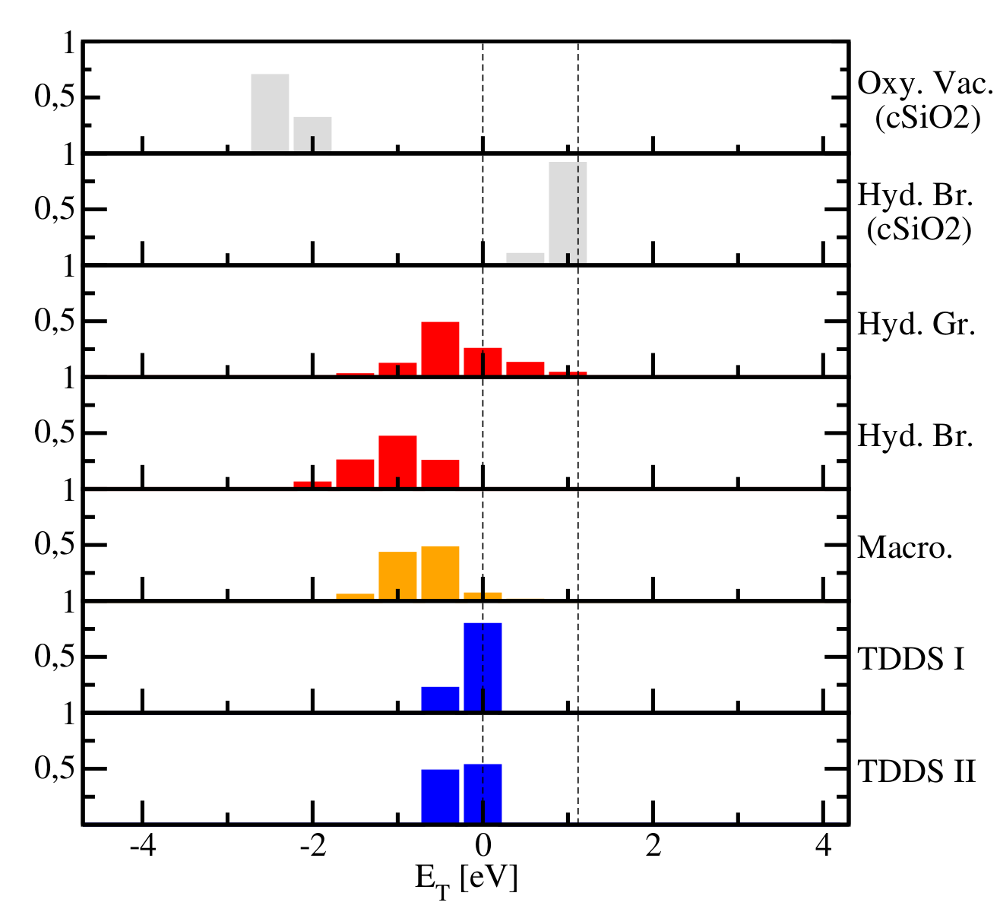
Fig. 1: Histogram of the defect levels for the hydroxyl E' center (Hyd.Gr.) and the hydrogen bridge (Hyd.Br.) contrasted to the values extracted from the degradation curves (Macro.) and TDDS.
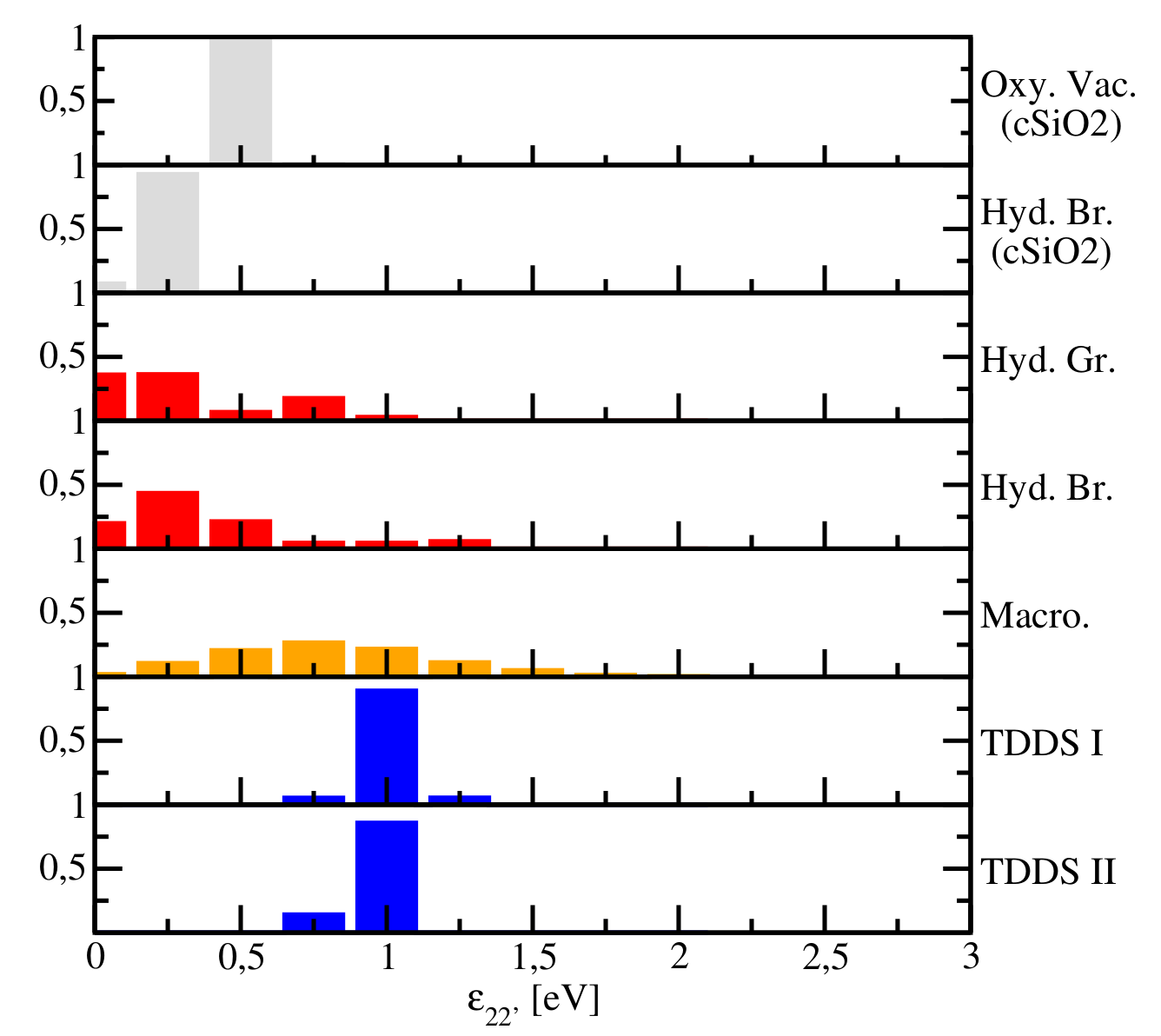
Fig. 2: The same as in Fig. 1 but for the energy barrier between the two stable configurations in the multi-state NMP model.