![]() |
|
Biography
Paul Ellinghaus was born in 1986 and grew up in Pretoria, South Africa, where he completed his Bachelor of Electronic Engineering, at the University of Pretoria, in 2008, whereafter he worked as a Research Engineer for the university spin-off company, INSiAVA, for 9 months. He completed his Master studies in Mathematical Engineering, within the Erasmus Mundus MathMods consortium, in L'Aquila (Italy) and Nice (France), in 2011. Thereafter, he joined the Process Integration group at IHP Microelectronics, in Frankfurt (Oder), Germany, as a Research Assistant for 15 months. He joined the Institute for Microelectronics in January 2013 to support research efforts relating to the Wigner-Boltzmann equation. He completed his doctoral degree, related to this topic, in February 2016 and is currently a post doctoral researcher at the Institute.
Simulation of Time-Resolved Quantum Transport with Scattering for Novel Nanoelectronics
The continuous performance gains brought about by the scaling of "traditional" semiconductor devices, primarily logic transistors and memory cells, will soon be over, as we approach their long-predicted physical limits. The inevitability of this fact provides the impetus for research in nanoelectronics to explore other avenues where performance is to be gained by using novel device concepts. The advent of engineering with quantum mechanics promises to open up many new design possibilities using concepts such as superposition or entanglement. In this pursuit, suitable simulation tools will gain further prominence and play a fundamental role in understanding and exploring the feasibility of applying oftentimes counterintuitive concepts to design new devices.
The Wigner Ensemble Monte Carlo (WEMC) simulator, part of the open source ViennaWD project, is now capable of simulating the time-dependent quantum evolution of wave packets (electrons), while also taking scattering into account. The ability to simulate two-dimensional structures over timescales approaching picoseconds affords the possibility of utilizing the WEMC simulator to investigate physical problems. It is important to investigate the capability of studying time-resolved transport topics such as the oscillations appearing in highly miniaturized circuits where classical theory fails. The consideration of scattering in quantum transport is also essential when it to comes to studying the decoherence of qubits (entangled quantum states) that are a fundamental building block for quantum computing.
The mesoscopic ring-like structure, represented in Fig. 1, has been simulated using the WEMC simulator to demonstrate some quantum effects. Fig. 2 captures a moment in the evolution of a wave packet that "splits" between the branches of the ring. An interference pattern is formed as the wave packets meet again at the exit of the ring. Fig. 3 shows the effect a lower potential energy in the left branch of the ring (Fig. 1) has on the interference pattern. This is essentially the gated Aharonov-Bohm effect, which has been demonstrated experimentally in steady-state operation. Insight into transient dynamics is lacking, however, and can be provided by numerical simulation.
Current research is directed at investigating the effect of potential perturbations on the interference pattern at other locations both inside and outside the conduction channel. This could ultimately be used to devise new sensor concepts for high-sensitivity measurements.
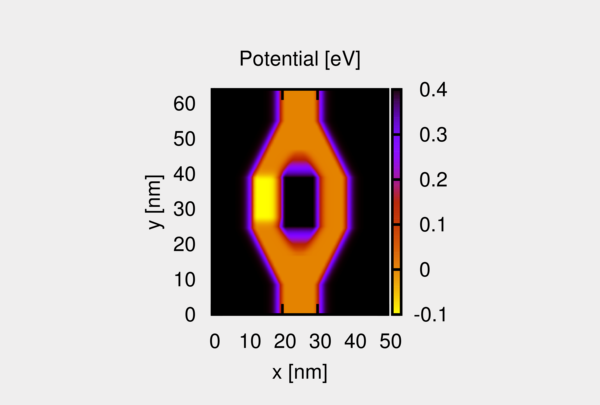
Fig. 1: Potential profile of a mesoscopic ring structure with a lowered potential in the left branch (achieved by gating).
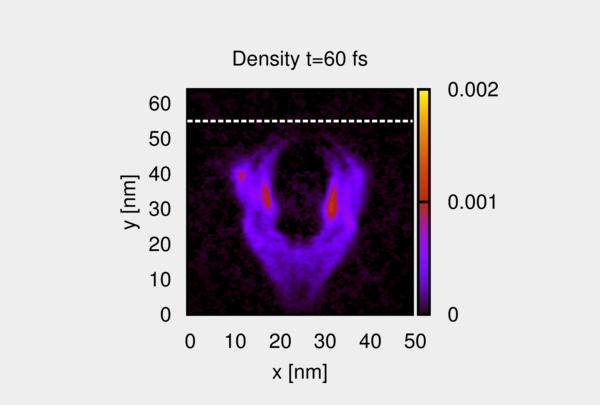
Fig. 2: Probability density of a single wave packet after 60 fs of evolution, moving from bottom to the top of the structure in Fig. 1.
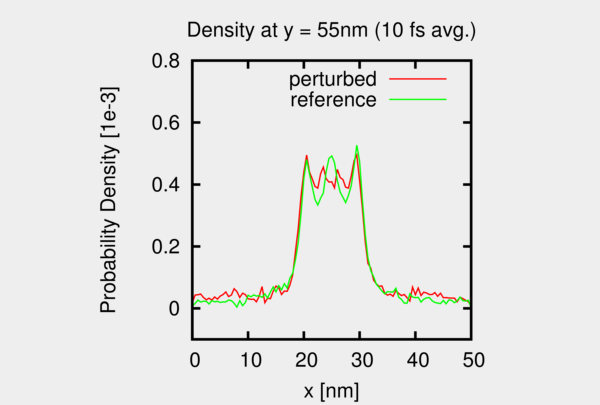
Fig. 3: Comparison of the interference pattern after 120 fs, formed along the dashed line in Fig. 2, with and without a perturbation, i.e. an additional potential energy drop of 0.1 V on the left branch.