![]() |
|
Biography
Viktor Sverdlov received his MSc and PhD degrees in physics from the State University of St.Petersburg, Russia, in 1985 and 1989, respectively. From 1989 to 1999 he worked as a staff research scientist at the V.A.Fock Institute of Physics, St.Petersburg State University. During this time, he visited ICTP (Italy, 1993), the University of Geneva (Switzerland, 1993-1994), the University of Oulu (Finland,1995), the Helsinki University of Technology (Finland, 1996, 1998), the Free University of Berlin (Germany, 1997), and NORDITA (Denmark, 1998). In 1999, he became a staff research scientist at the State University of New York at Stony Brook. He joined the Institute for Microelectronics at the Technische Universität Wien, in 2004. In May 2011 he received the venia docendi in microelectronics. His scientific interests include device simulations, computational physics, solid-state physics, and nanoelectronics.
Silicon Spintronics
A breathtaking increase in the performance and speed of modern integrated circuits has become possible thanks to the constant miniaturization of CMOS devices. Growing technological challenges and soaring costs are gradually bringing CMOS scaling to an end, however, intensifying the search for complementary or even alternative technologies and computational principles. Spintronics has therefore attracted a great deal of interest because of its potential to build novel spin-based devices that may become superior to today's charge-based elements.
One such promising device is a SpinFET, in which the current through the channel depends also on the electron spin polarization, providing new opportunities to create reconfigurable logic. Its on-current depends on the relative orientation of the ferromagnetic source and drain magnetizations, while the possibility of manipulating spins in the channel offers an additional parameter through which one can control device performance.
In contrast to charge, spin injected into a non-magnetic material gradually decays, due to spin-flip processes, to its equilibrium zero spin value. Thus, reducing spin relaxation to ensure a spin lifetime sufficient for application remains a challenge. In order for SpinFETs to become a reality, the injection, detection, propagation, and manipulation of spins in silicon by purely electrical means must be realized. Spin injection is achieved by pumping an electric current through a tunnel contact from a ferromagnetic contact to silicon.
Non-equilibrium spin accumulation in silicon must result in a voltage signal measured between a ferromagnetic and normal electrode. It turns out that the amplitude of the signal extracted from this measurement is orders of magnitude larger than expected. It is determined by spin-dependent magnetoresistance due to trap-assisted resonant tunneling (Fig. 1) and not by spin accumulation.
Spin dynamics at the trap determine the magnetoresistance. At room temperature, the effects due to finite spin coherence and lifetime must be taken into account. This is done by introducing corresponding relaxation terms into a Lindblad equation for the density matrix evolution of a spin on a trap. As expected, spin relaxation suppresses the amplitude of magnetoresistance, but strong dephasing leads to an unusual decrease of the magnetoresistance half-width in the perpendicular magnetic field (Fig. 2). Results are promising for a new generation of magnetic field sensors.
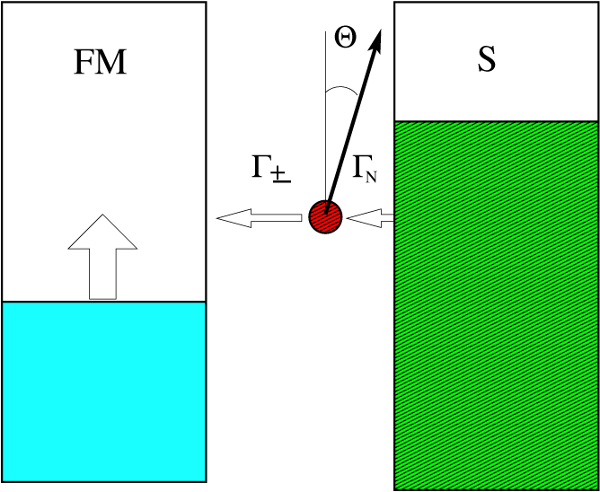
Fig. 1: The trap between the ferromagnetic (left) and normal electrodes. The magnetic field, B, is not aligned with the magnetization in the ferromagnet.
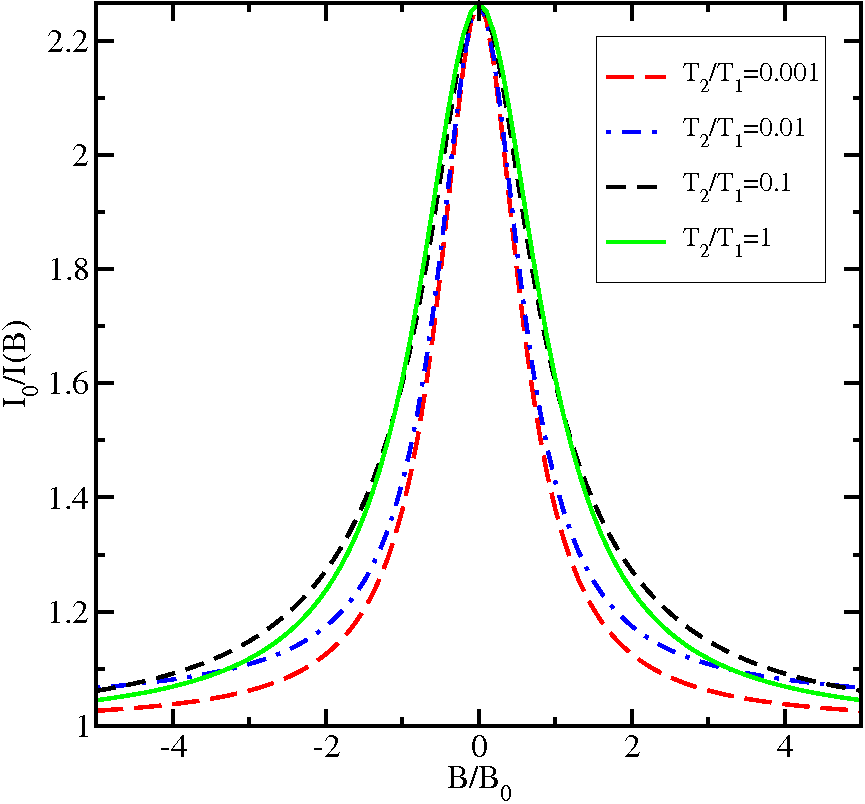
Fig. 2: Magnetoresistance as a function of the perpendicular magnetic field for p = 0.8. The field, B0, is assumed parallel to the magnetization in the ferromagnet.