![]() |
|
Biography
Markus Jech was born in Vienna in 1986. He received the degree of Diplomingenieur in Physics from the Technische Universität Wien in 2014. In January 2015 he joined the Institute for Microelectronics, where he is currently working on his doctoral degree.
The Physics of Hot Carriers in MOSFETs
One of the main reliability issues in modern MOS devices is hot carrier degradation. Although we have been aware of this detrimental phenomenon for decades, its underlying physics are not yet fully understood. It is widely accepted that hot-carrier damage is due to broken Si-H bonds at the Si-SiO2 interface, so-called Pb-centers, triggered by channel carriers. These Si dangling bonds are electrically active with an amphoteric character, meaning they have two trap levels inside the Si bandgap. When charged, they locally perturb the device's electrostatics and degrade the mobility.
Up to now, very little has been known about the actual defect creation mechanism. It is believed that two physically different but interacting mechanisms cause the hydrogen desorption. These two processes are called multi vibrational excitation (MVE) and the antibonding (AB) mechanism. The former is attributed to a vibrational excitation of the Si-H bond, triggered by a subsequent interaction with channel carriers, while the latter is related to an electronic excitation of one of the bonding electrons. In the field of surface chemistry, these processes are known as dissociation induced by multiple electronic transitions (DIMET) and dissociation induced by electronic transition (DIET).
To reveal the underlying physics of these processes, we performed a thorough density functional theory study. We started with small molecules and clusters, SiH4 and Si4H10, to calculate potential energy surfaces (PES) for different spin states of the Si-H bond. The results for different spin and charge states, namely singlet for the ground state, triplet for the electronically excited state and doublets for the ionic states of the Si-H bond, are shown in Fig. 1.
One can clearly see that the equilibrium positions are shifted with respect to the ground state PES. Additionally, one can conclude that the transition from the singlet to the triplet state at the equilibrium position of the Si-H bond would require at least 7.6 eV (this value depends on the molecule size). Thus, the AB process, described as an electronic transition, can be ruled out as a possible desorption mechanism of hydrogen, since carriers do not have such high energies in modern scaled devices.
Quite interestingly, however, both ionic doublet states show a bonding character with equilibrium positions shifted in respect to the ground state PES. For the negatively and positively charged state, we considered the case where the 6σ* orbital was occupied by an electron and the 5σ orbital was occupied by a hole. Stokbro et al. calculated the energy level of these two orbitals for a Si slab passivated with H. Their results are summarized in Fig. 2 in the context of an Si-SiO2 interface of a MOS device.
Combining these results, one can conclude that hot carriers at the interface can tunnel into these resonances and form temporary ionic states. If the carrier flux is high enough, hot carriers can transiently occupy these resonances and thereby trigger hydrogen dissociation. This explanation is fully consistent with the description of DIMET processes used in surface chemistry.
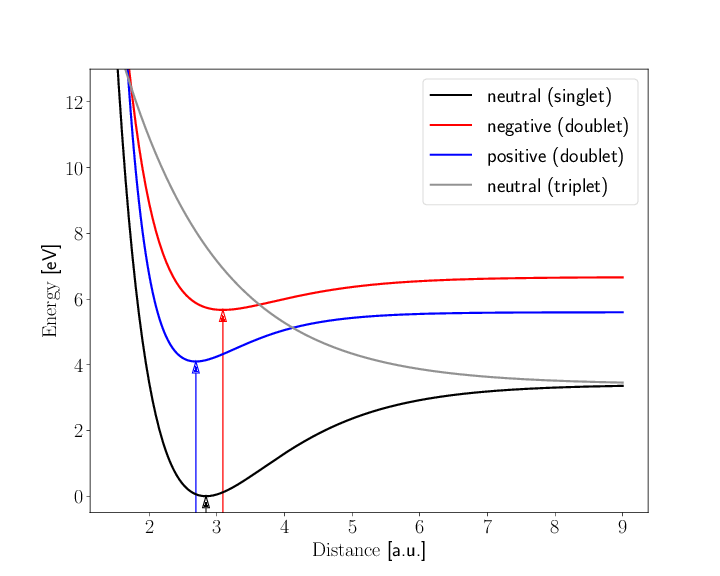
Fig. 1: Different charge and spin states for the silicon hydrogen bond.
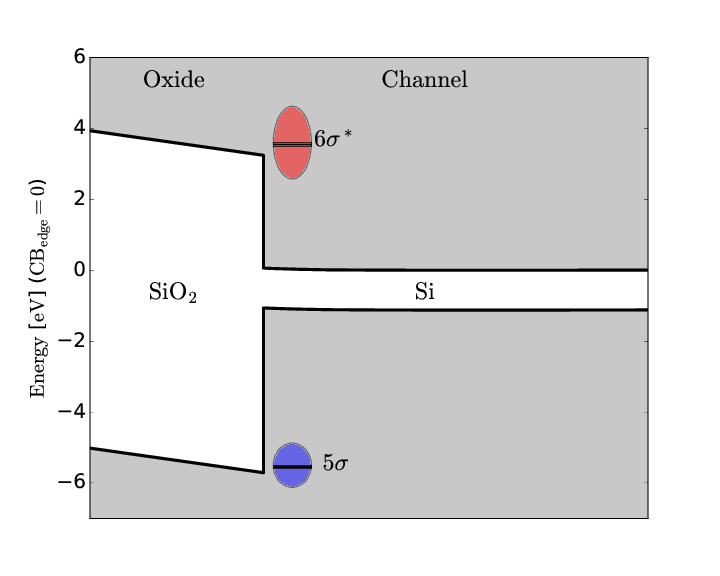
Fig. 2: Energetical positions of the bonding and antibonding orbital in an Si-SiO2 environment.