![]() |
|
Biography
Markus Kampl was born in Vienna, Austria, in 1988. He studied electrical engineering at the Technische Universität Wien, where he joined the Institute for Microelectronics in October 2012. In November 2015 he received the degree of Diplomingenieur. Since that time he is working on his doctoral degree.
Advancing the Investigation of Hot-Carriers by Improving the Vienna Monte Carlo Simulator
We have improved the Vienna Monte Carlo Simulator (VMC) in order to advance investigation into hot-carrier effects in MOS devices. For this purpose, we implemented electron-electron (e-e) scattering to model the high-energy tail of the distribution function more accurately. A novel averaging technique has been developed, which gives us more sampling values and has thereby reduced statistical errors. We further coupled the VMC with the drift diffusion simulator Minimos-NT (MMNT) so as to make it self consistent.
Literature shows that e-e scattering has a significant influence on the high-energy tail of the distribution function. Due to its complexity, this scattering mechanism is often neglected or roughly approximated, which leads to widely varying results. Most of these approximations show a common behavior: The probability of hot-carriers rises when e-e scattering is turned on.
In order to investigate whether this behavior is physical, we implemented an e-e scattering model in the full-band simulator VMC, with as few approximations as possible. Our results clearly showed an enhancement of the high-energy tail of the distribution, which however is not as pronounced as predicted by a deterministic Boltzmann solver, which relies on additional approximations (Fig. 1). In order to gain a better understanding of the e-e scattering process and the approximations used and in order to allow for additional comparisons, we also implemented this scattering mechanism for the analytical band structure model in VMC.
The most common averaging method for single-particle Monte Carlo simulation is the before scattering method. In this method, the averages are calculated from the values before a scattering event occurs. If there is a part of a device with few or no scattering events, as would be the case in near ballistic transport, there would be no data with which to build the mean values.
We implemented a novel technique whereby the averages are calculated every time the particle enters a new grid cell. If there is a part of the device where the average distance between scattering events is much larger than the size of an average grid cell, this technique provides results with reduced statistical error. In order to make the simulation self-consistent, we introduced an iteration loop until consistency was obtained, which calculates the mobility using VMC simulation and then recalculates the potential using MMNT.
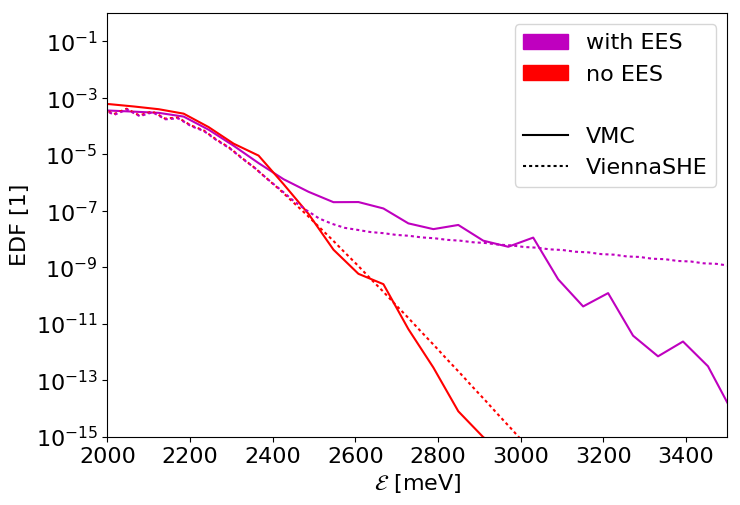
Fig. 1: High-energy tail of the energy distribution at the drain side of a 65 nm MOSFET channel.