![]() |
|
Biography
Viktor Sverdlov received his MSc and PhD degrees in physics from the State University of St.Petersburg, Russia, in 1985 and 1989, respectively. From 1989 to 1999 he worked as a staff research scientist at the V.A.Fock Institute of Physics, St.Petersburg State University. During this time, he visited ICTP (Italy, 1993), the University of Geneva (Switzerland, 1993-1994), the University of Oulu (Finland,1995), the Helsinki University of Technology (Finland, 1996, 1998), the Free University of Berlin (Germany, 1997), and NORDITA (Denmark, 1998). In 1999, he became a staff research scientist at the State University of New York at Stony Brook. He joined the Institute for Microelectronics at the Technische Universität Wien, in 2004. In May 2011 he received the venia docendi in microelectronics. His scientific interests include device simulations, computational physics, solid-state physics, and nanoelectronics.
Spin-Dependent Tunneling in Magnetic Structures
The breathtaking increase in performance and speed of modern integrated circuits is continuously supported by the constant miniaturization of CMOS devices. Due to transistor leakages, however, rapid growth in dynamic and stand-by power has become a pressing issue. The microelectronics industry is facing major challenges related to power dissipation and energy consumption, and microprocessor scaling will soon hit a "power wall".
A promising way to overcome this trend is to develop non-volatile memory technologies. The development of an electrically addressable non-volatile memory, combining high speed and high endurance, is essential to achieving these goals. Employing non-volatility in the main computer memory, as a replacement for conventional volatile CMOS-based DRAM, is particularly promising. To further reduce energy consumption, replacing caches (SRAM) in the modern hierarchical multi-level processor memory structure with non-volatile memory technology is essential.
Tunneling magnetoresistance, a transport phenomenon that arises due to spin-dependent charge properties, has revolutionized the way information is stored, preserved and recovered from modern non-volatile magnetic memory devices, like hard drives and spin transfer torque MRAM. Large magnetic-field-dependent responses detected recently in light-emitting diodes are caused by the correlated spin-charge dynamics. Magnetic-field dependent spin-correlated trap-assisted tunneling is also responsible for the unusually large amplitudes of signals in experiments on three-terminal electrical spin injection into non-magnetic semiconductors. This is due to the fact that the electron with its spin anti-aligned (spin-down) to the ferromagnetic contact magnetization has difficulty escaping from the trap due to the much smaller spin-down density of states, while another electron cannot enter the trap due to the Coulomb blockade. Even without the Coulomb blockade, however, trap-assisted tunneling through a ferromagnet dielectric normal-metal structure depends strongly on the magnetic field.
We considered the tunneling transport through a quantum well between two ferromagnetic contacts with arbitrary magnetization orientations in a magnetic field (Fig. 1). The dependence of the magnetoresistance on the magnetic field is shown in Fig. 2 for several angular arrangements. The linear part of the dependences is suitable for magnetic field sensing. The field magnitude that can be detected is determined by the tunneling rates between the quantum well and the contacts and thus can easily be engineered. The constant built-in stray field along the favorable direction can be adjusted by placing the well appropriately between the two non-colinear ferromagnetic contacts.
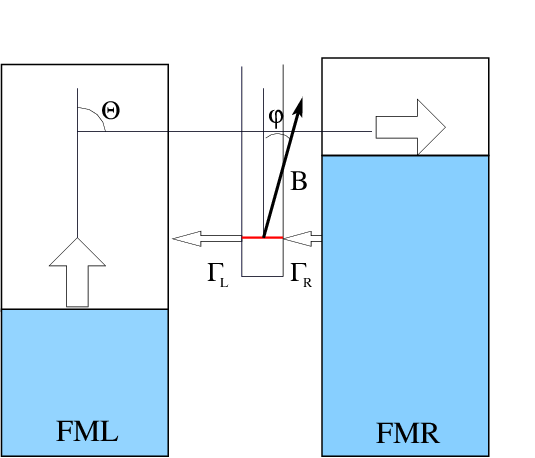
Fig. 1: The quantum well is positioned between the two ferromagnetic non-collinear electrodes. ΓL and ΓR are the tunneling rates and B is the magnetic field.
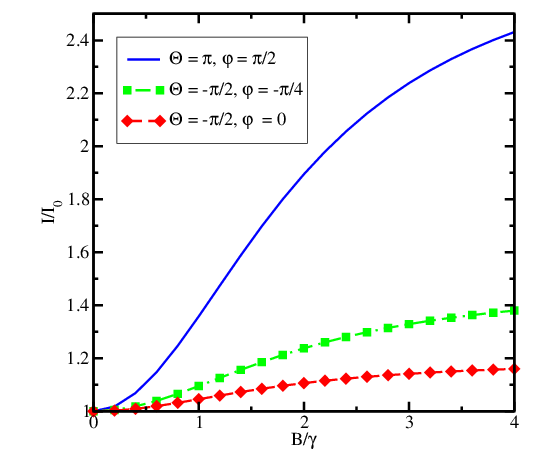
Fig. 2: The dependence of the magnetoresistance on the magnetic field for several angular arrangements. The anti-parallel contact arrangement results in a stronger dependence on the magnetic field orthogonal to the magnetizations.